An international team of the Event Horizon Telescope (EHT) project, including members from API, has announced the first results at the press conference on last Wednesday (Streaming link). The team has revealed the first ever image of the supermassive black at the heart of M87, a galaxy within the Virgo galaxy cluster, 55 million light years from Earth. This monumental image offers the glimpse of what is really going on near the event horizon, the point beyond which material (including light) never escape from the gravity of the black hole.
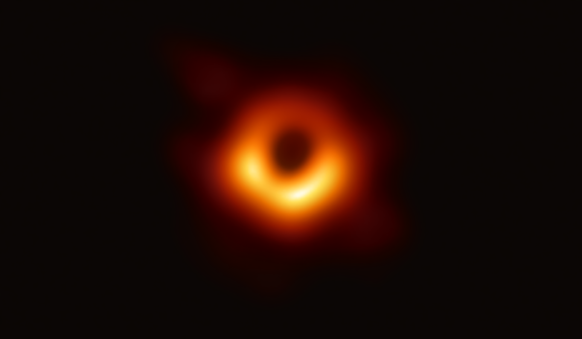
By definition, a black hole itself is literally dark as it gobbles everything around it. However, under certain circumstances, it has been expected that an outline of the black hole (i.e. event horizon) could be seen by a disk of glowing gas. In fact, what we witnessed was the meal time of the black hole.
It is believed that the status of the accretion disk around the black hole varies depending on the accretion rate (See the figure below). In today’s post, I will briefly describe which type of dining table the black hole favors under the certain circumstance.
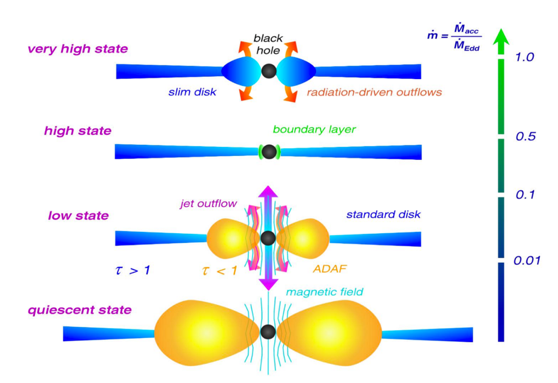
In theoretical perspectives, the accretion flow can be divided into two classes broadly: cold accretion disk and hot accretion flow. While cold accretion disk is optically thick and geometrically thin and occurs at relatively high accretion rate, hot accretion flow is optically thin and geometrically thick (H/R ~1, where R is radius and H is thickness of the disk). In general, the hot accretion flows are thought to be a typical disk structure around the black hole in low-luminosity active galactic nuclei (AGN), including our own Sgr A* (center of Milky way galaxy) and M87, which was the target of EHT observation.
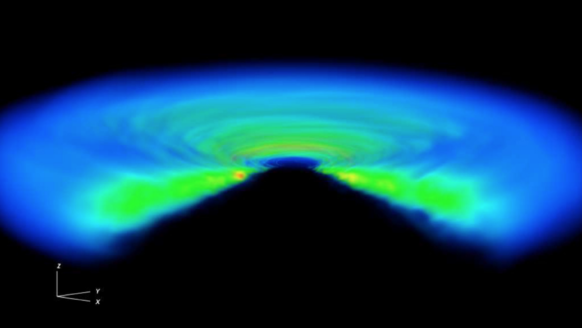
Cold and thin disk model (at high accretion rate)
The first theoretical model of an accretion disk was a thin disk model, which was developed in the early 1970 (Novikov & Thorne 1973, Shakura & Sunyaev 1973). The gas temperature in this model lies in the range 104-107 K. Since this range of temperature is quite lower than the virial temperature, which is a mean temperature in the gravitationally bound system, the accretion disk has a thin structure geometrically. In this model, a rotating flow can move inward along the accretion disk as a consequence of viscous transport of angular momentum such as magnetic rotational instability or disk winds. This cold accretion disk radiates mainly thermal blackbody-like radiation and has successfully explained many luminous active galactic nuclei (AGNs).
Hot accretion flow model (at low accretion rate)
This model was invented to explain the hard X-ray emission seen in some black hole sources. Unlike the cold disk model, the gas temperature of the gas in this model is much higher and close to the virial temperature. In such a high temperature, the plasma is known to have a different temperature between the ions and the electrons because the Coulomb interaction becomes inefficient. The essential ingredient of this model is that the viscously dissipated accretion energy would go into heating the accretion flow, which makes the disk geometrically thick. In this regime, the energy would advect inward rather than radiate away because the cooling time scale becomes significantly longer dynamical time scale. The general class of the hot accretion solution is called ”Advection-Dominated Accretion Flows (ADAFs)’’ (Narayan & Yi 1994). This solution has been invoked to explain the observed features in low-luminosity AGNs, including Sgr A* in our galactic center.
Slim disk (at very high accretion rate; super-Eddington rate)
When the accretion rate exceeds the Eddington limit, where the outward radiation pressure is balanced with the inward gravitational force, the accreting gas becomes optically too thick to radiate all the dissipated energy. As a result, radiation is then trapped and advected inward. This model looks similar to ADAFs in a sense that ”advection’’ plays an important role and the radiative efficiency is very low, but the reason of the ”advection’’ is different and occurs at the different accretion regime; slim disk occurs at very high accretion rate and ADAFs occurs at low accretion rate (see the figure). This model has been applied to narrow-line Seyfert galaxies and Ultra-luminous X-ray sources.
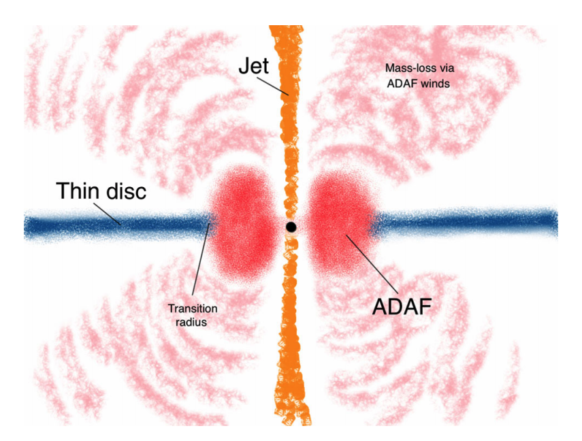
Structural transition of the accretion disk
In fact, most low luminosity AGNs have a two-zone accretion flow, which consists of a thin disk at large radii and a hot accretion flow at small radii. This is called the truncated disk model (see the figure above). If the accretion rate increases, the truncation radius becomes small and the thin disk extends all the way down to the inner stable circular orbit when the accretion rate approaches the critical value.