In today’s post I will talk about Compton scattering – a physical process by which light and matter interact. Interestingly, this process is responsible for colouring our sky and also for generating electromagnetic radiation from the most energetic phenomena in the universe.
A Brief History
This phenomenon was first discovered by Arthur H. Compton in 1923, for which he won the Nobel Prize in 1927. This process was also an important evidence of the particle nature of light, i.e, it proved that light (or electromagnetic radiation) exhibits particle like behaviour. In other words, if light only behaved like waves then the energy lost by light during Compton scattering could not be explained (see this link for more details about the dual nature of light).
Compton Scattering
Compton scattering is a physical process by which light (or particles of light called photons) interact with matter (to be specific charged particles, such as the electron). This scattering process can be imagined as a collision between a photon and an electron, similar to a collision between two balls (e.g. billiard balls, this analogy is a good way to start thinking about it most of the time, but not always as we will see). Just like in a billiard ball collision, energy can be exchanged between the photons and electrons during this collision.
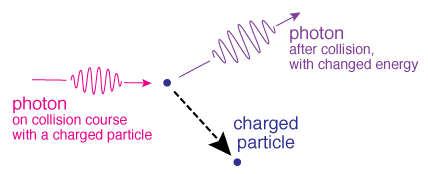
In Compton scattering, a photon collides with an electron at rest. In terms of the billiards analogy, this collision can be thought of as one between a ball at rest (the electron) and a moving ball (the photon). During the collision, the photon imparts some energy to the electron and as a result the electron is no longer at rest (see Figure 1). The photon correspondingly loses some energy, and now travels along a direction so that the total momentum of the system is conserved. Thus after Compton scattering, the electron at rest, gains energy from the colliding photon.
There is one important difference between the colliding billiard balls and photon-electron collision. In collisions between billiard balls, if we know how the balls collide before the collision, we can predict with 100% accuracy, how fast and in which direction the balls will travel after the collision. However, in case of electron-photon collisions, the directions of travel after the collision cannot be determined with 100% certainty. We can only talk about the probability of finding a photon traveling in a certain direction after such a photon-electron collision, as the photon is more likely to be scattered in some directions than others. In other words, imagine several identical electron–photon pairs, all set up to undergoing Compton scattering. Even if the setup is identical for all these different pairs, after the collision, the electron-photon pairs will be travelling along different directions and with different energies (we would obtain a distribution of directions / energies).
Terrestrial Implications
Blue Origin of the Sky
The blue colour of the sky was first explained by Lord Rayleigh in 1871 as the scattering of sunlight by tiny particles (much smaller than the wavelength of light). Sunlight is made of several colours (ranging from red, orange… green, indigo, blue etc). One can associate each colour of light to a specific wavelength or energy. What Lord Rayleigh discovered was that the amount of sunlight scattering in the Earth’s atmosphere depends upon the light’s colour or energy. The higher the light’s energy the more it will be scattered. This means is that light having lower energy (such as red or green) will be scattered (or bounced around like billiard balls) less than higher energy light (eg. blue), which will scatter the most.
It turns out that Rayleigh scattering is the same process as Compton scattering when the light (or photons) have low energies! At low energies, the scattering process causes very little change in the light energy but changes the direction of motion – hence the term scattering! The Compton scattering process is fundamentally responsible for the blue color of our sky.
So why is the sky blue? Due to differing energies the constituent colors of sunlight are scattered differently, with blue being scattered the most. Blue light is scattered in different directions, over and over again, and it diffuses across the entire sky. That is why when we look up at an arbitrary point in the sky (unless we stare directly at the sun which we should not!), we see blue light from that has been redirected into our line of sight due to many scatterings.
Cloudy Skies
This can also explain why cloudy skies aren’t blue. Clouds are composed of water droplets which are larger in size than the air molecules. Unlike air molecules, these larger sized particles scatter light more or less equally irrespective of its colour. As a result, all components of sunlight are scattered almost equally with no colour being given a preferential treatment, resulting in white / grey skies.
Red Sunrises / Sunsets
At dawn or dusk when the sun is near the horizon, sunlight has to travel through a larger part of the atmosphere to reach us. Low energy light (such as red) reaches us straight from the sun as it does not scatter much. However, blue light traveling through this increased atmospheric distance is scattered so much more that it diffuses out of the line of sight. This makes sunsets and sunrises appear reddish in colour.
Turning the process on its head: Inverse Compton Scattering
Energizing photons
A video illustrating how a low energy photon (red colour) can scatter off an electron and become energized into an X-ray photon (represented by blue colour). Video Credit: NASA
Previously we talked about how a photon collides with an electron at rest and gives up part of its energy. Now let’s make things more exciting, by colliding a moving electron and a photon (which Einstein has told us – always travels at the speed of light). Things get interesting now as the collision can transfer energy to the photon from the electron. This is the converse of Compton scattering, and is thus called inverse Compton scattering.
The analogy of two colliding billiard balls breaks down here. If the electrons go faster and faster (i.e., they become relativistic), quantum mechanical effects come into play. These effects decrease the probability that an electron will collide with a photon (the same effects emerge if the photon has energy comparable to the rest mass of the electron).
Astrophysical Implications: High Energy Photons
As we have seen, inverse Compton scattering can transfer energy from electrons to photons. If electrons have a lot of energy (for example, they are extremely hot or travelling really fast) and have photons in their vicinity, they will undergo inverse Compton scattering and give up some of their energy to these photons. The electrons are thus ‘cooling down’ by giving up energy to this photon ‘coolant’. These energized photons may travel to Earth, and once detected can reveal the conditions of the source that energized them (e.g., temperature of the electrons etc). This process helps us probe several astrophysical objects such as – astrophysical jets or outflows in X-ray binaries, Active Galactic Nuclei, and Gamma-ray bursts, interaction of cosmic microwave background radiation (CMB) with hot gas in galaxy clusters etc.