When you stand on the top of a hill, and shout your name, after a short delay, you will recursively hear your bellow, although your voice is successively fainted. The returned sound is an echo. You might be curious, why cannot we hear an echo in a room? This is because the human ear cannot distinguish echo from the original sound if the delay is less than 0.1 seconds. The speed of sound through air is approximately 343 m/s, therefore, the reflector (the walls of a room) must be placed more than 17.2 m away from the sound source (a man who is also a recevier here) for the echo to be differentiated.
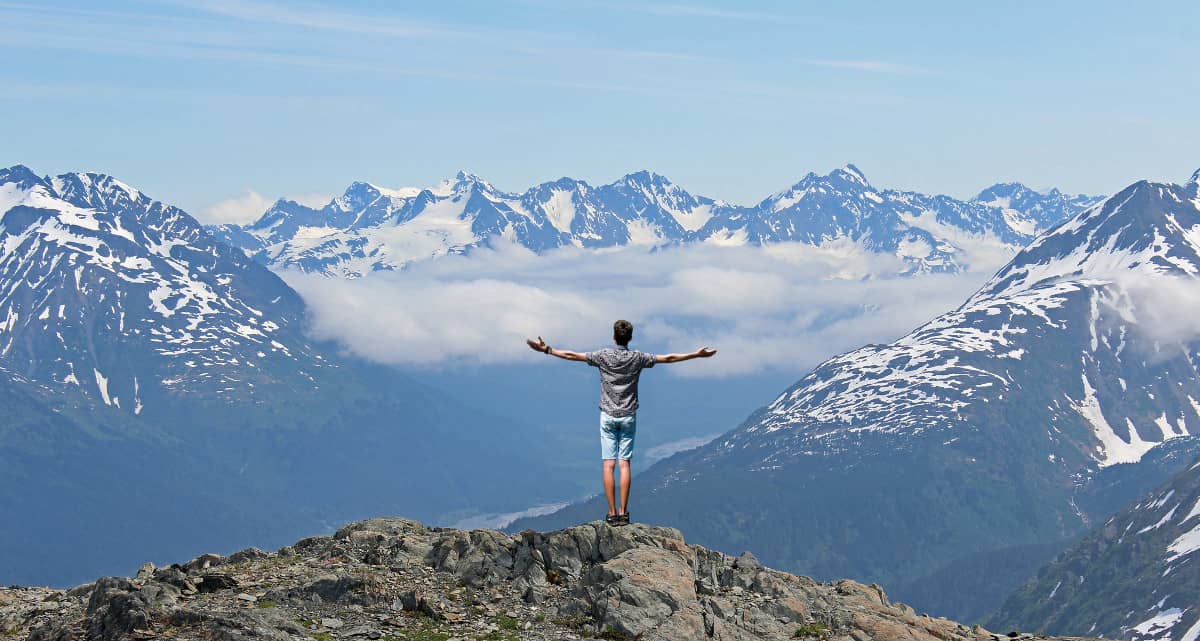
The property of an echo depends on the structure of the reflector. For simplicity, we can image a ring-like wall and a man (sound source) stands at the center. When the man shouts his name, after a while, he can only hear his name once because any part of the wall has the same distance to the man, and echoes from different parts of the wall are fully overlapped.
Image the man starts running towards the wall. When he moves a bit off-centre, he still hears his name once. But this time, the pronunciation of each syllable in his name will be elongated as those echoes from different regions are slightly separated. When he moves further off-centre, those echoes gradually no longer overlap. In theory, he is able to simutaneously hear every single syllable of his name, but technically, he hears nothing but faint noise which lasts relatively longer as the echo returned back becomes chaotic and elongated.
Given sound wave and the structure of the reflector, we know what kind of echo received. On the contrary, once we know what is the echo like, we could explore the structure of the reflector or the sound wave. In fact, that is exactly what we do in astronomy! In scientific research, sometimes it is not possible to observe the source directly due to significant absorption or that the source turns off and doesn’t radiate anymore. However, if the source is surrounded by a huge amount of gas/dust, we could infer the activity of the source at present or in the past via the echo from its ambient medium. In this post, I will show you two kinds of echoes in astronomy.
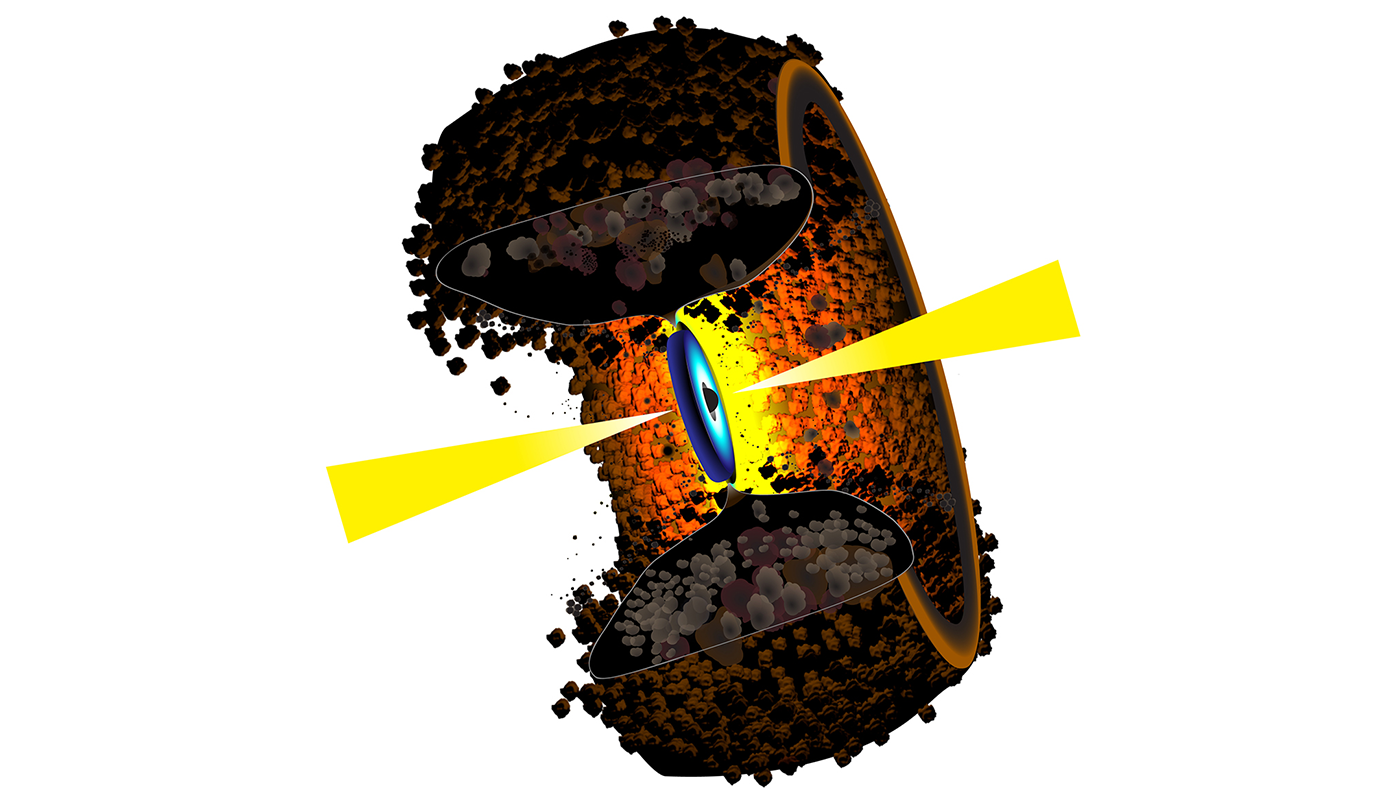
Infrared echo when a supermassive black hole eating a star:
Black holes are the most powerful engines in the universe (for more details, see Yixuan’s post: Black Holes, the most Powerful Engines in Nature). When a star passes by a supermassive black hole (SMBH), it could be disrupted by tidal force of the SMBH and nearly half of its mass will be accreted onto the SMBH resulting a flare peaking at optical or ultraviolet frequency so that we can detect this event using an optical telescope. Nevertheless, the host galaxy of this SMBH could be extremely dusty, especially for the core region where SMBH is located, a dust torus could form (see Figure 2). The dust will significantly (even fully) absorb the optical/ultraviolet radiation from the central SMBH and re-emit it in infrared band. Instead of an optical outburst, we will see an infrared echo! Typically, the duration of a primary outburst ranges from few months to few years while the infrared echo could last from few to ten years (the duration of the signal will be elongated as shown in sound echo).
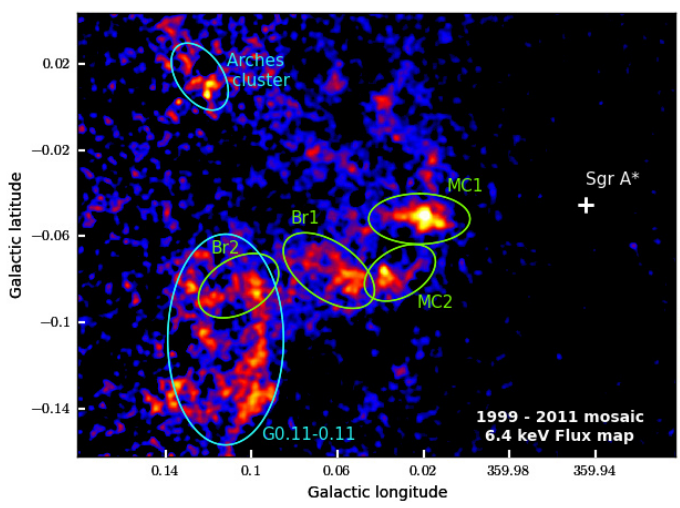
Iron Kα line emission echo from Sgr A* in the past:
Sagittarius A* (Sgr A*) is the SMBH in our Milky Way and is also one of the least luminous SMBHs we have known. Although Sgr A* is extremely quiescent nowadays, it could be much brighter in the last few hundreds of years. There are many molecular clouds (MCs) located a few hundred light years away from Sgr A* and emitting strong iron Kα line emission which is believed to be the result of interaction between high-energy photons from an outburst of Sgr A* in the past and the neutral iron atoms in MCs (see Figure 3). Although it is not possible to directly detect this outburst any more, we could reconstruct it through the locations of the MCs and the iron Kα line echo! Many studies show that Sgr A* used to be 3-4 orders of magnitude more luminous than it is now.