In my previous blog post, I introduced the General-relativistic Radiative Transfer Code, which is an important tool for computing the radiative properties from simulated results (see “a bridge between observation and numerical simulation”). Today, I would like to provide some examples of how the image of black hole shadow, photon ring and the spectrum would change depending on the different physical properties, some of which are very difficult to be constrained yet.
1. Black hole image in different frequency
The well-known monumental image of the M87 black hole shadow, which was announced in 2019, was captured at 230 GHz by the telescopes in the Event Horizon Telescope array. The intrinsic size of the emission from M87 (and Sgr A* in our Galaxy) is smaller with higher observation frequency (see Movie 1 below) because the optical depth of the synchrotron emission becomes smaller (i.e., more transparent). The frequency 230 GHz is high enough to observe the inner accretion flow in M87 (and Sgr A*). In addition, interstellar media in the Galactic center cause scattering, which blurs the image. The blurring is more severe for lower frequencies, which makes us catch details of the accretion flows with radio observations.
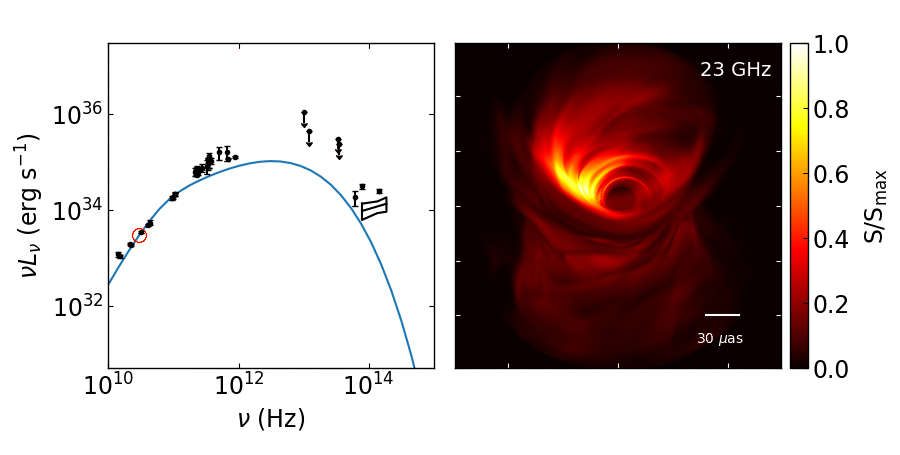
2. Black hole image in the different viewing angle
M87, the first target for the black hole imaging, has a beautiful large-scale jet. Assuming that the black hole spin axis is aligned with the jet (while this assumption should be examined for the possible mis-aligned jet (see “Warps and Twists around Black holes”)), it has been widely accepted that the viewing angle between the line-of-sight and the black hole axis would be small (< 30 degrees; note that the EHT adopted the angle of 163 degrees, which is ~17 degrees from the axis). However, for Sgr A* in the center of our Galaxy, the viewing angle is highly uncertain due to the absence of such a jet.
Movie 2 shows how the image would look different as the viewing angle changes from face-on (theta=0) to edge-on (theta=90) for Sgr A*. In general, the fluxes slightly increase (see the left plot) with increasing viewing angle mainly due to the orbiting plasma that is approaching the observer and the emission being more strongly Doppler boosted at a higher viewing angle. In the image, the left edge of the ring gets brighter with a higher viewing angle because the plasma rotates toward the observer there.
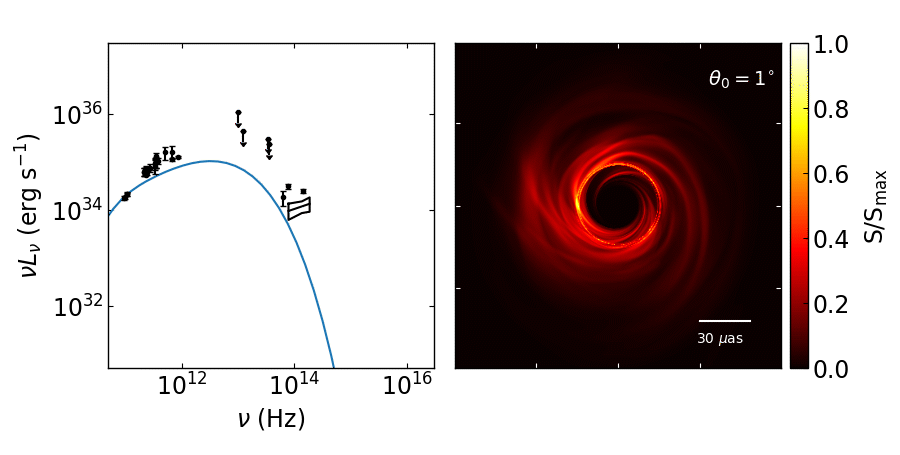
3. Black hole image in the different electron temperature modeling
For computing the radiative property from numerical simulation, one of the largest uncertainty is what the electron temperature is. Since dynamics is governed by heavy elements (l.e., proton), we obtain the proton temperature from simulation, but we need to make some approximation to infer the temperature of electron, which is less dominant for dynamics but is very important for radiative processes (See the blog “electron microphysics in GRMHD simulations” for the detailed introduction).
There are several prescriptions for calculating the electron temperature, in which the temperature ratio between ions and electrons would be determined by physical properties of plasma, such as magnetic field strength. These prescriptions helped people to explore the emission around the black hole from simulated data.
Movie 3 shows how the spectra and image look different with the different values of R_high in R-beta prescription (Moscibrodzka et al 2014). This prescription was designed that lower R_high derives higher electron temperature at the disk. In other words, as the value of R_high becomes higher, the emission from the disk is relatively weaker.
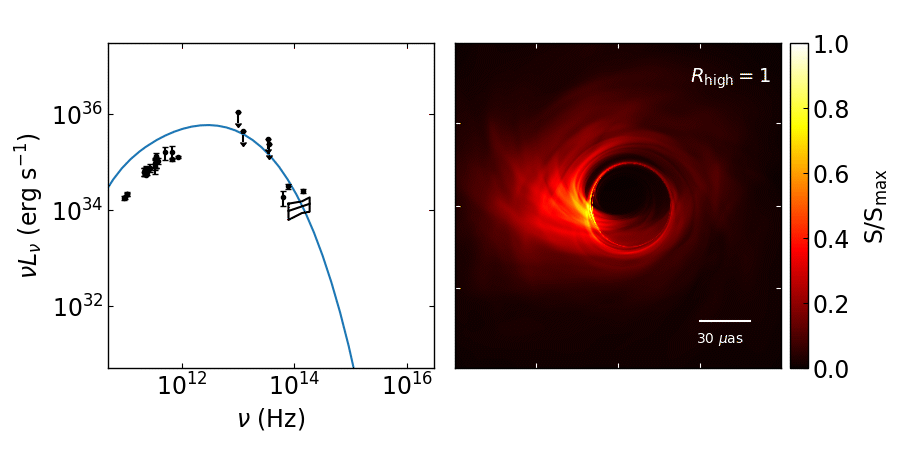
In summary, there are still many uncertainties that impede us to catch the underlying physic of accretion flows around the black holes. However, the collaborative efforts in observation and theoretical modeling will help us understand the nature of plasma near the black hole, by better constraining plasma properties and developing more physically motivated models.